Genes
We are interested in understanding how molecules in our brain influence neural activity and behavior. We use the fruit fly Drosophila melanogaster to study this due to amazing spatiotemporal resolution for visualizing and manipulating genes, RNA and proteins in the brain.
Circuits
We are interested in understanding how neurons influence behavioral choice and how alcohol and drugs of abuse influence this. We combine in-depth anatomical connectome data with intersectional genetic tools to map circuits for reward, memory and behavioral decisions in the Drosophila brain.
Behavior
We are fascinated with interested in understanding the molecular basis of behavior, and are pushing the boundaries of the types of complex behaviors we can measure in Drosophila. We combine computer vision and machine learning approaches to determine high-content behavioral tracking of flies learning, remembering and making decisions.
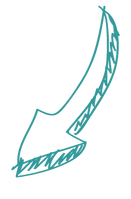

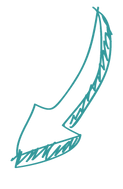
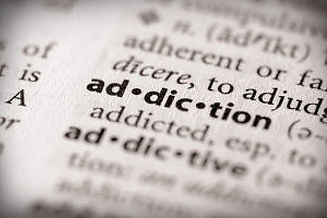
Understanding the mechanisms of addiction
Why do we crave alcohol and not apples? We are innately wired to seek and respond to rewards, but addictive substances can overwhelm our natural reward pathways resulting in intolerable cravings and reduced impulse control. The consequence is prioritization of drug seeking above all else, creating turmoil in the lives of people and their communities worldwide.
Until recently, we had relatively little understanding of how addictive substances influenced the molecules in the brain. An explosion in knowledge of molecular genetics has revealed that psychoactive substances including drugs and alcohol drastically affect which genes get turned on and off in the brain, and therefore which molecules are present to fuel brain cell function. These mechanisms are highly conserved across species, all the way from flies to humans. This tells us there is something fundamental about how addictive substances influence our natural reward mechanisms to cause disease, underscoring the neurobiological basis of addiction.
We strive to understand how alcohol and drugs of abuse influence gene expression in the brain to result in long-lasting cravings. Our ultimate goal is to discover more effective treatments for alcohol and drug use disorders. The work we do is also answering fundamental questions about how memory is encoded in the brain.
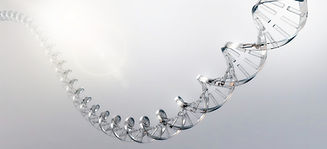
Understanding dynamic gene regulation in the brain
Our brains are made up of more than 86 billion neurons and 85 billion glial cells, and the diversity of different neuron and glia types is astounding. Indeed, we are just beginning to understand the vast diversity in anatomy and function of the cells in the brain. This diversity is dependent on which genes get expressed in each cell, and the timeline for gene expression. Recent findings from flies, mice, rats, monkeys and humans demonstrate the gene expression is a highly dynamic process and can change with how the animals are treated and how they react to this treatment. Genes get transcribed to mRNA, and mRNA then gets translated to proteins, which drive the function of the cell. Thus, when gene expression changes, the proteins that make up the cell change and can alter cell function. This can result in changes in neuronal plasticity, and consequently, behavior.
The beauty of this dynamic nature is that it demonstrates the plasticity of the brain at the molecular level, and consequently at the neuronal activity level. It also suggests that while our genes affect how our brain develops, expression of these genes in our daily lives is dynamic and adaptable to suit our needs. We are working on understanding how alcohol and drugs of abuse affect dynamic gene expression in memory circuits in Drosophila melanogaster. We combine genetics, transcriptomics, epigenetics, molecular biology and biochemistry to understand how natural rewards and addictive substances alter gene expression, and how that influences behavior. We are particularly interested in understanding how addictive substances mediate alternative splicing and the functional significance of these changes.
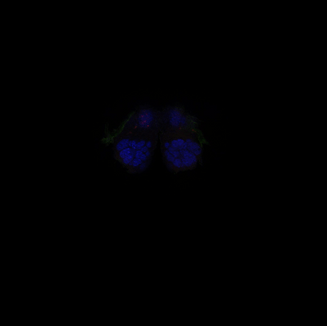
Understanding circuit dynamics in the brain
In order to understand how reward is processed and drives behavioral decisions such as drug seeking, we need to better understand how neuromodulators regulate cellular function at the level of individual circuits and their component cells in behaving animals. Whereas mammalian models lack tools that permit this type of cellular specificity, our work on a complex but accessible network of dopamine, octopamine, serotonin, acetylcholine, glutamate, GABA neurons in the Drosophila mushroom body can reveal circuit dynamics that will be useful for understanding the function of similar circuit motifs in more complex brains.
We defined a simple circuit model that provides a framework for understanding the sequence of events through which dopamine mediates alcohol reinforcement and ultimately drives alcohol seeking. Using a combination of molecular genetic, in vivo imaging, and behavioral approaches we define how circuits function during: i) a change in internal state due to intoxication of addictive substances, ii) formation and expression of sensory memories associated with addictive substances, and iii) operant self-administration for natural and drug reward. This work provides a unique opportunity to define, with unprecedented resolution, how circuits work during formation of memories associated with craving and motivated response.